The runaway greenhouse effect on Venus
What the science says...
Select a level... |
![]() |
![]() | |||
Venus very likely underwent a runaway or ‘moist’ greenhouse phase earlier in its history, and today is kept hot by a dense CO2 atmosphere. |
Climate Myth...
Venus doesn't have a runaway greenhouse effect
"I bought off on the “runaway greenhouse” idea on Venus for several decades (without smoking pot) and only very recently have come to understand that the theory is beyond absurd." (Steve Goddard, WUWT)
At a glance
Earth: we take its existence for granted. But when one looks at its early evolution, from around 4.56 billion years ago, the fact that we are here at all starts to look miraculous.
Over billions of years, stars are born and then die. Our modern telescopes can observe such processes across the cosmos. So we have a reasonable idea of what happened when our own Solar System was young. It started out as a vast spinning disc of dust with the young Sun at its centre. What happened next?
Readers who look up a lot at night will be familiar with shooting stars. These are small remnants of the early Solar System, drawn towards Earth's surface by our planet's gravitational pull. Billions of years ago, the same thing happened but on an absolutely massive scale. Fledgeling protoplanets attracted more and more matter to themselves. Lots of them collided. Eventually out of all this violent chaos, a few winners emerged, making up the Solar System as we now know it.
The early Solar system was also extremely hot. Even more heat was generated during the near-constant collisions and through the super-abundance of fiercely radioactive isotopes. Protoplanets became so hot that they went through a completely molten stage, during which heavy elements such as iron sank down through gravity, towards the centre. That's how their cores formed. At the same time, the displaced lighter material rose, to form their silicate mantles. This dramatic process, that affected all juvenile rocky planets, is known as planetary differentiation.
Earth and Venus are the two largest rocky planets. But at some point after differentiation and solidification of their magma-oceans, their paths diverged. Earth ended up becoming habitable to life, but Venus turned into a hellscape. What happened?
There's a lot we don't know about Venus. But what we do know is that the surface temperature is hot enough to melt lead, at 477 °C (890 °F). Atmospheric pressure is akin to that found on Earth - but over a kilometre down in the oceans. The orbit of Venus may be closer to the Sun but a lot of the sunlight bathing the planet is reflected by the thick and permanent cloud cover. Several attempts to land probes on the surface have seen the craft expire during descent or only a short while (~2 hours max.) after landing.
Nevertheless, radar has been used to map the features of the planetary surface and analyses have been made of the Venusian atmosphere. The latter is almost all carbon dioxide, with a bit of nitrogen. Sulphuric acid droplets make up the clouds. Many hypotheses have been put forward for the evidently different evolution of Venus, but the critical bit - testing them - requires fieldwork under the most difficult conditions in the inner Solar System.
One leading hypothesis is that early on, Venus experienced a runaway water vapour-based greenhouse effect. Water vapour built up in the atmosphere and temperatures rose and rose until a point was reached where the oceans had evaporated. In the upper atmosphere, the water (H2O) molecules were split by exposure to high-energy ultraviolet light and the light hydrogen component escaped to space.
With that progressive loss of water, most processes that consume CO2 would eventually grind to a halt, unlike on Earth. Carbon dioxide released by volcanic activity would then simply accumulate in the Venusian atmosphere over billions of years, creating the stable but unfriendly conditions we see there today.
Earth instead managed to hang onto its water, to become the benign, life-supporting place where we live. We should be grateful!
Please use this form to provide feedback about this new "At a glance" section. Read a more technical version below or dig deeper via the tabs above!
Further details
Venus may have experienced a runaway greenhouse effect in the geological past. To use the term 'runaway' is to refer to a highly specific process when discussed by planetary scientists. Simply having a very hot, high-CO2 atmosphere is not it. So let's start with a tutorial on Venus at the present day.
Venus’ orbit is much closer to the sun, which means it receives almost twice the solar radiation at the top of its atmosphere than Earth. Venus also has a very high albedo which ends up over-compensating for the closer distance to the sun. The result is that less than 10% of that incident solar radiation reaches the surface. High albedo can be attributed to sulphur-bearing compounds, along with minor water vapour (around 20 ppm). These substances form globally encircling sulphuric acid-dominated cloud decks (fig. 1). Venus’ atmosphere also has a surface pressure of around 92 bars (or if you like, 92,000 millibars), equivalent to what you’d feel on Earth beneath more than a kilometre of ocean.
Fig. 1: Venus in its shroud of clouds - a false colour composite created by combining images taken using orange and ultraviolet spectral filters on the Mariner 10 spacecraft's imaging camera.The images used to create this view were acquired in 1974; the RH one has been enhanced to bring out texture and colour. Image: NASA.
Observations of the water vapour content in the Venusian atmosphere show a high heavy to light hydrogen isotopic ratio (D/H). This is best interpreted as the product of a preferential light hydrogen escape to space: deuterium escapes less easily. Venus is considered to have had at least 100 times its current water content in the past (e.g. Selsis et al. 2007 and references therein).
The greenhouse effect on Venus today is primarily caused by CO2, although water vapour and SO2 are important as well. Since most of the radiation that makes its way out to space comes from only the very topmost parts of the atmosphere, it can look as cold as Mars in infra-red (IR) imagery. In reality, the surface of Venus (Fig. 2) is even hotter than the dayside of Mercury, at a deadly 477 °C (890 °F).
Like Earth, the Venusian clouds also generate a greenhouse effect. However, they are poor IR absorbers and emitters compared to water clouds. The sulfuric acid droplets forming the clouds can also scatter IR radiation back to the surface, producing another form of the greenhouse effect in that way. In the dense Venusian CO2-rich atmosphere, there are IR-handling processes at work that are unimportant on modern Earth.
Fig. 2: The Soviet Union's Venera 14 probe captured two colour panoramas of Venus's surface in 1982. This panorama came from the rear camera. Image: Russian Academy of Sciences. More images can be seen at: https://www.planetary.org/articles/every-picture-from-venus-surface-ever
How to get a Runaway?
To get a true runaway greenhouse effect on Venus, you need a combination of solar radiation and the presence of a greenhouse gas. That gas has two key requirements. It must be condensable and it needs to be in equilibrium with its surface reservoir. In addition, its concentration must increase with temperature, as explained by the Clausius-Clapeyron relation. For Venus to enter a runaway greenhouse state, the greenhouse gas of interest is water vapour, plus its liquid reservoir, the water making up the oceans.
The greenhouse effect on any planet involves impeding the flux of outwards longwave radiation to space. Water vapour is very good at this so can potentially lead to a positive feedback runaway scenario. That works as follows: higher temperatures cause ever more water to evaporate and then drive temperatures even higher and on and on it goes - while there is an available liquid water reservoir.
Through water vapour's effectiveness at blocking IR, the outward longwave radiation flux eventually flatlines. If the incoming Solar flux is constantly greater than that outgoing flatline value, the planet is tipped out of radiative equilibrium and we have that runaway. If you like, it has a fever. The reservoir for water vapour - the oceans - is vast. That means the system may only be able to return to radiative equilibrium once the runaway process has stopped. In the extreme runaway greenhouse effect, that cessation may only happen at the point when the whole ocean has evaporated.
On present-day Earth, there is a strong temperature inversion, called the tropopause. It is situated between the troposphere and stratosphere. You can see it on thundery days when the tops of storm-clouds spread out beneath it to form the familiar anvil-shapes. The tropopause thus forms an effective barrier to moisture getting into the stratosphere. At the height of the tropopause on Earth, in any case, it's already too cold for water to remain in the vapour phase. The wispy clouds making up thunderstorm anvils consist of ice crystals. This impediment to water vapour's ascent is often referred to as a 'cold trap'.
In a runaway scenario, such as that proposed for Venus, no such impediment exists. This means the upper atmosphere would have become moist too. On Venus, the troposphere extends to a much greater height than on Earth. There is no stratosphere - we're talking about a very different situation here. That is critical because water vapour, upon reaching such great heights, has energetic Solar ultraviolet (UV) radiation to contend with. The UV is effective at splitting the H2O molecule into its constituent elements. Once that has happened, the hydrogen in particular is easily lost to space. One can envisage that once a runaway greenhouse effect got going, Venus' water content got steadily depleted in this manner through time. If Venus ever had oceans, they must have evaporated into oblivion. Because of the 'cold trap', this form of water-depletion is of very little significance on Earth - thankfully.
Once that water was lost, the chemical processes that lock up carbon in rocks on Earth could not operate. All of them involve water somewhere. Thereafter, every addition of carbon to the atmosphere, large or small, stayed up there. Most CO2 was probably of volcanic origin. The result was the 96.5% CO2 atmosphere and hellish surface temperature of Venus today.
Earth and the Runaway: Past and Future
Currently, Earth is well under the absorbed solar radiation threshold for a runaway greenhouse effect to occur. Its water condenses and is recycled back to the surface as rain, rather than accumulating indefinitely throughout the atmosphere. The opposite is true for CO2, which builds up and up through our emissions, only checked by natural removal processes. Note here that the runaway greenhouse threshold is largely independent of CO2 since the IR opacity is swamped by the water vapour effect. This makes it difficult to justify concerns over a CO2-induced runaway on Earth.
However, this immunity to a runaway greenhouse effect will not last forever. The most realistic scenario for Earth entering a runaway occurs a few billion years in the future, when the sun's brightness has substantially increased. Earth will then receive more sunlight than the outgoing longwave radiation escaping to space. A true runaway greenhouse effect is then able to kick in. Caveats apply, though. For example, greater cloud cover could increase planetary albedo and delay this process.
Interestingly, some (e.g. Zahnle et al. 2007) have argued that Earth may have been in a transient runaway greenhouse phase within the first few million years of its existence. Geothermal heat and the heat flow from the moon-forming impact would have made up for the difference between the net solar insolation and the runaway greenhouse threshold. But if this happened it could only have lasted for a relatively short period of time - since we still have plenty of water on Earth.
For further reading, a recent review paper (Gillmann et al. 2022) explores the various hypotheses concerning the evolution of the Venusian atmosphere over geological time. There's also an excellent book chapter (Arney & Kane. 2020, currently available as a PDF at arXiv). As might be expected, difficulties in fieldwork are plural on Venus and designing a probe that survives touchdown and can go on to do the required data-collecting is still some time away. The key piece of evidence we need to confirm the existence of a runaway greenhouse effect in deep time would be for free water having once been present. But it is apparent that large parts of the surface were covered with lava flows from monster volcanoes at some point. Is that evidence nowhere to be seen, or is it just hiding? Time will tell.
Last updated on 21 January 2024 by John Mason. View Archives
@ 149
From your link to Wikipedia:
So, as long as T is constant and the gas is confined (V is constant), then, yeah.
For the rest of us, PV = nRT
[RH] Edited out post @150 rather than delete (per request) in order to preserve the numbered comment reference used in this thread.
@149
Mike Hillis
"Heat stays the same but T goes up".
This statement is a nonsense. If heat in the gas (actually it is more accurate to talk about the 'internal energy' of the gas) doesn't change, temperature cannot change. Because temperature is the direct measurement of some of that internal energy.
The following article on Wiki has a good discussion of the Thermodynamic (or Kinetic) definition of Temperature.
The eqution relating the internal energy of a gas, actually the kinetic energy of translation of the molecules in the gas, is as follows:
E = 3/2 kBTk
where:
E is the mean kinetic energy of a molecule in the gas, in Joules
kB = 1.3806504(24)×10−23 J/K is the Boltzmann constant
Tk is the kinetic temperature in kelvins (K)
Temperature is directly proportional to the internal energy of the molecules.
Temperature is the measurement of their internal energy.
So saying heat doesn't change but temperature does is nonsensical. Temperature is the measurement of 'heat'.
So if the amount of heat doesn't change, temperature cannot change, by definition.
If the amount of heat does change, temperature must change, by definition.
Next, this comment.
"The equation states that product of pressure and volume is a constant for a given mass of confined gas as long as the temperature is constant.
So, as long as T is constant and the gas is confined (V is constant), then, yeah.
For the rest of us, PV = nRT"
Mike if the temperature is constant (T is unchanged), then the internal energy hasn't changed. If the mass is constant (n is unchanged) then nRT is constant. So PV is constant, whether confined or not.
What can happen when gas parcels are unconfined is that internal energy might change - T changes. Or mass might change - n changes. Without one of those changes PV is still constant.
But when we are talking about bulk parcels of air in the atmosphere, there is very little mixing and it occurs relatively slowly. So any change in n is minimal. And similarly, without mixing into a parcel, convection cannot transfer much heat quickly into a parcel. Coinduction is a very very weak heat transfer mechanism in gases. And in the Troposphere where the air is 'optically thick', radiation is a poor energy transferer. So the assumption of Adiabatic processes is actually quite good. Heat transfer into a parcel is minimal.
So vertically moving parcels of air essentially can't change n, essentially can't change internal energy through heat transfer since things are adiabatic. So nRT is constant so PV is constant.
However, as they move to different altitudes they need to equalise pressure with the surrounding air. And this happens fairly quickly.
So P must change to equilibrate with the surroundings. OK, thats fine, V changes to match so that PV remains constant. But there is a problem. And your first item in your list highlights this:
"1. Parcel moves down and compresses".
The parcel can't compress itself! It has to be compressed by something outside it.
And that something is the surrounding air. Pressure equalisation means that the surrounding air compresses the parcel. PV would remain constant because everything is Adiabatic (see here for a definition of an Adiabatic Process) except that it is not an Isenthalpic Process.
There is energy transfer into the parcel!
This is not as a Heat Transfer - that satisfies the Adiabatic condition - but is an energy transfer into the parcel as work done on it. (See the definition of Work here)
The surrounding air has to perform mechanical work on the parcel to compress it. And this adds energy to the parcel. This then means the internal energy of the parcel has increased. T has increased! So PV can increase.
This is why descending air masses warm up. They are compressed by the surrounding air and this adds energy to them, increasing their temperature.
And the reverse applies to ascending air masses. Pressure equalisation means they have to do work on the surrounding air to expand and match pressures. Sp energy needs to be expended by the parcel to do that work. Since the process is Adiabatic, the only energy source available to supply the energy for this work is the parcels internal energy. So the parcels internal energy drops - it cools.
Mike
Lets consider a piston and cylinder with some air inside it. The air and the piston/cylinder are at a constant temperature.
Now I push the piston in, reducing the volume to half. If I do it quickly enough so there is no time for heat transfer between the air and the cylinder/piston then the process is essentially adiabatic.
So what should happen. No heat transfer, no mass transfer, so nRT is constant. So V is halved and P is doubled, and T doen't change surely.
But that isn't what happens. The work needed to compress the piston has to be added in. So the total internal energy of the air increases, T increases so nRT goes up. V is halved, and P increases by more than double! And we now notice that T has increased.
This often confuses people. They see the temperature increase and assume that the act of being more compressed is why T is higher.
It isn't.
T goes up because energy has been added due to the work needed to compress the piston.
There is a reverse case. If we allow the volume to double, P would be cut in half. Now if this expansion had to do work, then internal energy would be expended to do it, P would drop by more than half, and temperature would fall.
However, it is possible to contrive a situation where we can achieve this volume increase essentially without any work being done.
The setup looks like this.

A chamber is divided in two by a sliding door. On one side there is air at pressure P and temperature T. On the other side there is a vacuum. Everything is in thermal equilibrium, air and chambers.
Then we very rapidly slide the door away and allow the air to expand into the other chamber. No work needed to be done on the air to allow it to expand.
What happens?
Volume doubles, n is unchanged, P is cut in half, and T remains unchanged!
This is called Joule Expansion and was first demonstrated by James Prescott Joule in 1845 although others had known about it before hand.
There is actually a cooling of the gas that occurs but this is due to a secondary process, not related to the simple picture of PV = nRT we are discussing here. This secondary process is discussed at the end of the Joule Expansion article and also a related one on the Joule-Thompson Effect which is essentially about throttling processes.
Again the cooling seen in a real gas due to the JT Effect isn't intuitive so people can be misled and think that the act of being less compressed is the cause of the cooling. It isn't.
Next Mike, your comment to me and this comment &147.
"Yup. Only the sun can change the total heat content of the atmosphere.".
Of course thats true, but it isn't what we are discussing, or the point of my previous comment. We are discussing what can change the heat content of individual parcels of air, not the entire atmosphere. It isn't what changes the total heat content of the atmoisphere thar is at issue, it is the heat content at a smaller scale.
And at that scale there are processes that can change heat content of air parcels, I have described them in my previous comments.
There is a vertical movement of energy whan air rises and falls. Because the energy in an air parcel gets carried with it. So rising air carries haet to a higher altitude. Descending air carries heat to a lower altitude. Then pressure equalisation brings about a redistribution of that heat between the vertically moving air parcel and its surrpindings. And as a concequence, a vertical temperature distribution is created. This article about the Lapse Rate is worth reading. That is what it is all about.
So there is an engine that transports heat up and down until an equilibrium is established where the atmosphere has a vertical temperature profile based on the Lapse Rate for that planet and atmosphere. And if the atmosphere weren't in equalibrium, with one layer too warm or cold compared to other layers, this active pumping engine works to re-establish the profile.
So in the case of Venus, with most incoming solar energy being absorbed in the upper atmosphere, atmospheric mixing generates a substantial vertical temperature difference. And in the case of the Earth, where more incoming solar energy is absorbed at the surface, the same mixing generates a vertical difference as well.
Importantly, this only works when there is enough vertical movement and when the process is adiabatic. On Earth, this applies in the Troposphere but as we get up to the Stratosphere, these conditions breakdown. As the air thins, convection driving vertical movment drops right off, and as the atmosphere thins, radiation to space becomes a powerful factor, so things are no longer adiabatic. With substantial radiation loss to space, this trumps the weak Lapse Rate driver of circulation. So the temperature profile of the Troposphere is dominated by the Lapse Rate, but the temperature profile of the Stratosphere is dominated by radiative effects.
So in summary. You are right about there being a strong vertical heat pumping system but you are incorrect about the mechanism that drives it. Importantly, this mechanism is a heat distribution system. It is always working to balance amounts of energy at different altitudes to maintain a vertical temperature profile. So if energy is being added to the system at different altitudes from the Sun or tiny amounts from geotherml heat (or on Earth human energy usage) and at the same time being lost to space at other altitudes as infrared radiation, the Lapse Rate pump is continually moving this incoming heat around, trying to establish the temperature profile. And the net effect, after lots of moving around, is that the incoming energy is transported to the location that it needs to be at to get out to space. But the temperature profile of the entire optically thick, convective region of the atmosphere will be maintained by the pump, not just some parts of it.
So for Venus, where absorption and emission of radiation both largely happen at higher altitude, none the less the Lapse Rate pump will still ensure that lower levels in the atmosphere are hot enough to maintain the temperature profile.
So to a basic point, and the link you gave to the hockeyschtick site is an example of this.
There are a number of climate denier sites that make this sort of argument, with dubious thermodynamics, that the existence of the Lapse Rate in some way invalidates the Greenhouse Effect. The 'Venus isn't hot because of the GH Effect, it is hot because of the Lapse Rate' type arguments. What they all uniformly don't get is something simple.
The Lapse Rate is a part of the Greenhouse Effect!
There are 3 processes that work together to create the GH Effect.
1. Radiative Balance. The Earth has to radiate enough energy to space to balance the energy arriving from the Sun. If it doesn't radiate enough, heat builds up and everything fries, radiate too much and everything freezes. So 'the Earth' needs to be warm enough to generate the right amount of radiation to space. Too little and it warms until its right. Too much and it cools until it is right. Radiative Balance is always adjusting the temperature of 'the Earth' to keep it where it needs to be to match the flows.
Notice I have said 'the Earth'. Because actually it isn't the Earth, it is that part of the Earth where the radiation to space originates from. Radiation doesn't originate from 1000 meters down in the ocean for example. So Radiation originates from 'somewhere'. And Radiative Balance acts to manage the temperature of the 'somewhere'. Balance defines what that emission temperature is, but not where it comes from.
2. The presence or absence of GH gases in the atmosphere determines what altitude radiation to space originates from. More GH gases means it originates from hgher in the atmosphere. And if there were no GH gases present, then it would originate from the surface. GH Gases determine where the emission temperature is set by Radiative Balance.
For the Earth that is around 5 km up and the temperature is around -18 C. For Venus that is over 50 km up and -80 - -90 C. And if the amount of GH gases is changed, then the altitude changes. So if we added enough GH gases to raise the average emission altitude for the Earth from 5 km to 6 km, then the 6km level would then be driven to an average of -18 C by Radiative Balance.
3. Then the presence of the Lapse Rate pump re-balances the vertical temperature profile around the temperature at the average emission level. Because importantly, the Lapse Rate defines relative temperatures vs altitude, not absolute temperatures. It is Radiative Balance that sets that and the GH gases determine where. The Lapse Rate then drives the average temperature of the rest of the atmosphere column to fall into line with that.
So in my example, if GH gases increased the emissions altitude by 1 km, then that level would warm to an average of -18 C, and the Lapse Rate pump would then drive matching temperature changes through the rest of the air column. Including the surface. If the actual value of the Lapse Rate remained constant* (at -6.5 C/km), then adding enough GH gases to raise the emission altitude by 1 km would result in all parts of the air column warming by 6.5 C right up to the limits where the Lapse Rate pump works.
So the surface gets warmed because the upper atmosphere has to warm and atmospheric mixing propagates that up and down.
The Lapse Rate doesn't disprove the GH Effect. It is a part of it.
Sites like hockeyschtick, with their dodgy understanding, don't get this and end up spreading mis-information.
* Actually the Lapse Rate will change. In a warmer would there will be more evaporation and condensation. So the contribution from evaporation and condensation in determining the actual value of the Lapse Rate will be more important and the Lapse Rate will fall a little. This is called the Lapse Rate Feedback, it is a negative feedback, and offsets a little of the warming from more GH gases.
Glenn Tamblyn @153,
The gas equasion pV=nRT when stated as pV=constant does not define an adiabatic process. Ignoring any gravitational field, the equation is pVγ=constant where γ=Cp/Cv which for air =7/5. The reason for this is because the specific heat capacity at constant pressure (Cp) is not equal to the specific heat capacity at constant volume (Cv). As a result an adiabatic process changing p and V does involve a change in temperature.
Mind, I don't believe this is, as Mike Hillis @146 asserts, something a high school chemistry teacher would be immediately familiar with. And it is certainly far less worthy of criticism than the assertion Mike Hillis continues with @146:-
Firstly, pV=nRT says nothing about added heat or not adding heat. Secondly, applying work to a parcel of air says there is added heat, so such a process cannot be adiabatic. (While the potential energy change could be construed conceptually as outside the boundary of the system under consideration, raising or lowering p or V cannot.)
What is so odd about the position presented by Mike Hillis is that he is proposing what he describes as a Katabatic process which is a phenomenon born of the convection process and cooling at altitude. Yet Mike Hillis uses it to explain warming at altitude, the exact opposite to the convection process. Even the mash-up account he appears to be basing his nonsense on (Nikolov and Zeller's 2011 conference poster linked @122 above) and which overplays its use of the convection process still uses it in its usual direction. So I struggle to see where Mike Hillis is coming from.
Put very simply, any vertical movement of air in any atmosphere either takes heat from a higher altitude or adds it to a lower one. Is there vertical movement in an atmosphere? Of course. Must work be done to move the air up or down? Oh course, but that doesn't matter. All that matters is there is vertical movement. The fact that there is vertical movement proves that heat is being pumped downward. Even brownian motion does it.
The big question not answered here is Jupiter. How can there be a greenhouse effect on Jupiter when its atmosphere is made of H2? Answer, there isn't. The same thing happens on Jupiter as happens on Venus.
Venus' atmosphere is 93 bars. If you descend into Jupiter's atmosphere to a level where the density is 93 bars, the temperature is around 450 K according to
LINK
On that diagram, keep in mind there are 100,000 Pa to a bar so 93 bar is 9,300,000 Pa
[RH] Shortened link that was breaking page format.
MA Rodger
The Ideal Gas Law doesn't describe a process. It is a state law, so it describes a fixed state. Other things need to be considered to determine how and why the state changes. But the Ideal Gas Law will then describe the new state after a change.
So better to say that nRT is a constant if mass doesn't change and internal energy doesn't change. T isn't a measure of the total internal energy of a collection of molecules. It is a measure of the translational kinetic energy of those molecules.
They also have energy in rotational motion, internal vibrations, and potentially electron energy levels. Also variations in potential energy of the molecules as they move closer or farther apart. Gravity is one source of potential energy change as objects move apart or together. Electro-magnetic forcs produce similar. So too inter-molecular forces such as Van Der Waals forces. Then Quantum Mechanics also impacts this hugely, constraining possibilities.
So Specific Heat Capacity, measured at constant Pressure, or constant Volume, is essentially posing/answering the question 'how much added energy manifests as increases in molecular translational kinetic energy - temperature - as opposed to increases in other modes of energy storage."
Read this article at Wiki, looking at Degrees of Freedom etc.
So what is the basis for your statement that includes Cp/Cv. "As a result an adiabatic process changing p and V does involve a change in temperature."?
How are you determining that the total change in all internal energy forms - translational, rotational, vibrational, electron energy level, etc. - will result in a matching changes in only the translational component of energy - temperature.
Mike Hillis @147:
"Yup. Only the sun can change the total heat content of the atmosphere."
OK, let’s try to figure out the expected consequences of that:
As you know, Venus is closer to the sun than the Earth is (108.2 vs. 149.6 million km), but also has a much higher albedo (77% vs. 30.6% according to NASA’s fact sheet). When combining these two facts we find that each m2 of Venus (mostly its cloud tops) absorbs less than 2/3 as much solar energy as the Earth does.
So, if the total heat content in an atmosphere with a certain mass and composition is determined only by the amount of absorbed sunlight, we should expect the average temperature of Venus’ atmosphere as a whole – top to bottom – to be lower than the Earth’s, right?
Well, I tried to calculate a "mass-weighted" average temperature for both atmospheres based on the temperature and pressure at different altitudes.
The result for Earth was -22oC and for Venus.....+354oC !
Any adiabatic process can obviously be ruled out as an explanation for this, as the very cold but very thin upper layers of Venus’ atmosphere have way too little heat capacity to "compensate" for the heat in the much hotter and denser lower layers. Actually, when I in my calculation lowered the temperature to absolute zero above 30 km, it only reduced the average for the entire atmosphere to +309oC !
And again: The spectrum of outgoing IR measured by the Venera probe reveals very clearly that the high temperature isn’t caused by any kind of internal heat source, but the simple fact that almost all the heat from the surface and lower atmosphere is prevented from escaping directly to space.
Mike Hillis
"Put very simply, any vertical movement of air in any atmosphere either takes heat from a higher altitude or adds it to a lower one."
This statement is untrue Mike. Vertical movement of air downwards transfers heat, energy, downwards since the energy content of the moving parcel of air moves down with it. And a parcel of air moving upwards transfers heat, energy upwards.
So any vertical movement does move heat, but not all in the same direction. So any net movement of heat depends on the difference between thee two flows. And if the net of the two flows is zero, there is no effective flow.
What determines whether the net heat flow is up or down? Whether the vertical temperature profile matches the Lapse Rate. If the upper air is too warm, net flow of heat is downward, cooling the upper level and warming the lower. If the lower air is too warm it is a net upward heat flow, cooling the lower level and warming the upper level.
In near adiabatic conditions vertical air movement always generates a lapse rate.
Think about the logic of your idea that movement in either direction produces downward heat flow. If that we true, then heat would just continually build up and up in the lower level. Without radiation to provide an alternative means of loosing heat, the lower level would become impossibly hot, not merely 500 C or so.
As to your digression to Jupiter, vertical movement again explains the profile. If the depths of Jupiters atmosphere are optically thick, or on the case of Jupiter there are large quantities of clouds, then the adiabatic condition is met and a Lapse Rate can be created. There is no disparity.
And with clouds present, Jupiter will have a greenhouse effect to some extent. On the graph you linked to, note the clouds of different materials in the lower atmosphere, all contributing to a GH effect. Note also that some of the clouds are water, so there will also be water as vapour present - more GH effect.
Additionally, with some nitrogen present, there may be some GH type absorption and emission produced. This is due to what is known as 'collisionally induced absorption'. Molecules that aren't normally absorbers of IR radiation such as Nitrogen (N2) and Hydrogen (H2) can become transient absorbers and emitters during the finite time when they are colliding. This needs high densities and or low temperatures to make this possible, maximising the number and duration of collisions. So collisions between Nitrogen molecules on Titan for example allow N2 to create a GH Effect. And it has been theorised that collisions between N2 and H2 in the early atmosphere of the Earth may have contributed to the GH Effect then.
Mike
You might find this article interesting, discussing the structure of planetary atmospheres and the GH effect. Notice how Jupiter (and Saturn, Uranus & Neptune) all have lapse rates in their tropospheres.
Note also the discussion of likely gases in their atmospheres, including Ammonia and Hydrogen Sulphide, both GH gases.
And consider something else. A GH Effect doen't have to just depend on incoming sunlight. Any heat source that can add significant amounts of energy into an atmosphere which is optically thick in the infra-red and thus capable of being roughly adiabatic will produce a GH effect.
So in the case of the gas giants, they do have an internal GH effect because of clouds and GH gases, changing their inner tempeature due to the fact that they produce large amounts of heat internally. Whereas for the inner planets, internal heat is minimal but solar is significant - the heat source doesn't matter. So of all the planets, Mars has the least GH effect because:
Mike Hillis @158:
Ignoring for a moment the high cloud in the Jupiter atmosphere (which also generates a greenhouse effect), Jupiter's atmosphere contains 3000 ppmv of methane, 260 ppmv of ammonia, 6 ppmv of ethane, and 4 ppmv of water, all of which are greenhouse gases. The later two may be ignored, but the 3000 ppmv of methane and 260 ppmv of ammonia mean Jupiter's atmosphere is optically thicker for infra red radiation than is the Earth's. Specifically, at 1 bar atmospheric pressure, the infrared optical depth of the Earth's atmosphere is 1.9, while on Jupiter it is 6.3 (τ0 on Table 1 here). That in turn means the effective altitude of radiation to space for Jupiter (ie, the altitude where the optical depth equals 1) is closer to the tropopause for Jupiter (optical depth = 0.064) than it is for Earth (optical depth = 0.050).
Glenn,
I do not understand how energy can be transferred from a colder upper layer in the atmosphere to a warmer lower atmosphere. It seems to me a contradiction of the second law of thermodynamics.
The ocean forms a thermocline where circulation is restricted because the energy enters primarily from the top. I htought that the energy to circulate the atmosphere (even on Venus) comes from the sunlight absorbed by the surface and then transferred upward.
I have never an taken atmospheric science class so it may be some basic understanding that I lack. Can you provide a link that explains better how the lapse rate is maintained? I read the Wikipedia lapse rate article but I only understood what the lapse rate is and not how it is maintained in the atmosphere. I did not find much helpful on Google, but I do not know what to look for.
Michael Sweet:
Read Glenn's following text, and then let me try to explain it:
Glenn's statement applies to instantaneous transfer, not time-averaged. Energy content is a property of mass. If you move that mass in any direction, the energy it contains is moved with it. Upward movement of air is always moving energy upward; downward movement of air is always moving energy downwards. The exact vertical thermal energy flux is (heat content)*(vertical velocity). It does not matter - instantaneously - how the temperature of that air relates to air above it, below it, or around it. The simple movement of air moves energy.
Averaged over time, net mass transfer is zero (otherwise you'd create a vaccuum somewhere and an excess of air somewhere else), but net energy transfer is not. Net energy transfer depends on whether the upward moving air is - on average - warmer or colder than downward moving air.
This principle is actually used to measure the rate of sensible heat (thermal energy) transfer in the atmosphere, using a method called eddy covariance. The exact same method can be used to measure water vapour flux or CO2 flux (or any other measurable property), by using the commbination of humidity or CO2 concentration and vertical velocity. Water vapour flux is often used to determine surface evaporation, and vertical CO2 flux tells you about surface respiration/phiotosynthesis rates.
Although you must use very-fast-response instruments, so you can catch every puff, the necessary instrumentation is available off-the-shelf.
Since I've jumped in, I'll mention that wikipedia has an article on lapse rates.
There are three important lapse rates in earth meteorology:
One last comment for now. Mike Hillis has stated:
The is simply not true if you are talking direct heating, and you do not include oceans or land as part of the atmosphere. Most of the heating of the atmosphere comes from the surface, where most of the solar radiation is absorbed.
This post by Keven Trenberth provides details and graphics, such as this image:
The temperature structure of the stratosphere is driven by absorption of solar radiation (mainly UV), but the troposphere is dominated by energy gains from the surface in three forms:
You can argue that all that energy ultimately came from the sun, but it reaches the atmosphere indirectly. Ignoring that indirect path is not a particularly good idea, especially in a discussion of lapse rates, adiabatic processes, and the greenhouse effect.
[RH] Resized image.
Michael Sweet
Two points. Your first one about heat transfer from cold to hot is referring to the 2nd Law of Thermodynamics. And this can be a bit confusing. Firstly, the 2nd Law refers to Net heat flow, based on all the energy flows between a hot and cold point. More importantly, the 2nd Law applies to a closed system. It does not apply to an open system. So if we think of the system and ask what the boundaries are, they need to preclude flows from outside the boundary. The atmosphere isn't that, there are flows from the surface, flows in from the Sun, flows out to deep space, and even miniscule flows in from other stars and deep space.
Next, there are huge differences between the ocean and the atmosphere. Firstly, water is essentially imcompressible. So although pressure can increase hugely at depth very little actual compression occurs. So the density of parcels of water in the ocean doesn't vary by much; so much so that even changes in the salinity of sea water is a significant contributor to relativedensity change. And it is density differences that drives vertical convective movement.
The atmosphere on the other hand has huge density changes with altitude, with pressure. As a consequence, vertical movement is much easier, and any tendency to stratification is easily disrupted. Also the impact of coriolis forces is more profound. Since air velocities are much higher than ocean current velocities, change in location happens much faster, both horizontally and vertically. So more Coriolis force generated turbulence and vorticity effects. Next with its much lower density air is more easily able to move vertically since it takes much less energy to move a parcel of air vertically than the same size parcel of water. Horizontal air movements can more easily trigger vertical air movements , not just horizontal movements that infill the volume previously occupied. So turbulence and mixing can happen more easily.
So vertical movement is much easier. In the case of bottom heating which is how most heat flows into the Earths atmosphere, convection due to heating is easy to understand. However, even with top heating, which is more like what happens on Venus, mixing can still generate vertical movement. And some of the light from the Sun does penetrate to reasonable depth in Venus, enough to create some bottom driven convection.
Also, wind speeds on Venus are higher than on Earth, up to 700 km/hr in the mid levels. More speed, more turbulence.
So as long as there is anything that can generate reasonable vertical movements, in an optically thick, adiabatic situation, the Lapse Rate engine keeps running.
@ 168 Glenn Tamblyn
Thanks Glenn, looks like we're on the same page now.
Mike Hillis
Here is whats on that page.
Glenn, this is slightly off-topic but when speaking of emission elevations and temperatures, and optical thickness, you must specify wavelength. On Earth, emission of 15 micron IR occurs at the top of the troposphere at cold temperatures, but 9, 10, or 11 micron IR for examples, are emitted to space from Earth's surface (if not blocked by cloud) at very warm temperatures.
Mike, actually not off-topic, just a more detailed exploration of the topic. You might find this interesting
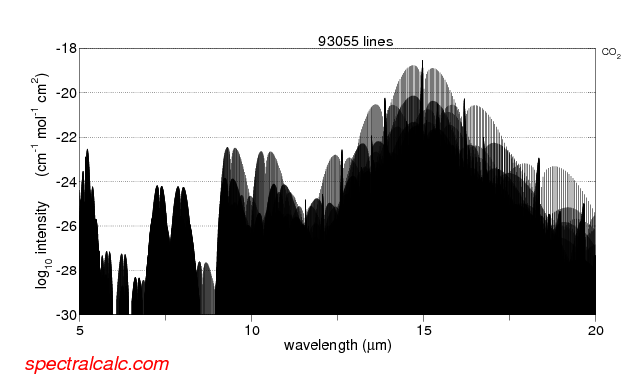
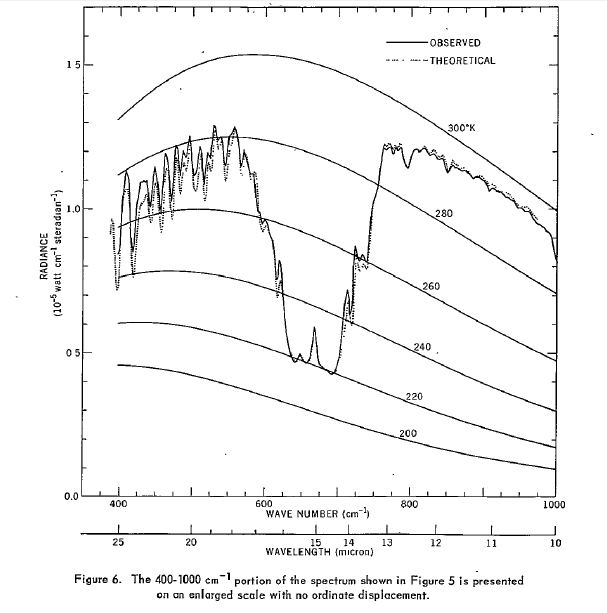
This is the data from the HiTran spectroscopic database for the Spectral Intensity of CO2 vs wavelength.
This is the starting point for evaluating absorption/emission strength. As you can see the vertical scale is logarithmic. The roughly triangular shape of the graph either side of 15 on a logarithmic scale is the primary basis of the logarithmic nature of CO2 forcing.
So peaks either side of 10 are also visible, and a peak near 5. However they are 4 orders of magnitude weaker than 15.
However this is only part of the picture. There is also a process called Line Broadening which, particularly in the lower atmosphere, smears out each line substantially. Extend this to Venus or Jupiter like atmospheres and individual lines can actually be smeared across much of this graph. There is more on this here.
As a result the actual spectrum observed by satellites is a product of some complex stuff.
Here is one such satellite measurement, from 1969.
That spike at 15 micron, at the centre of the CO2 'notch', doesn't just come from the top of the troposphere. It actually comes from the upper stratosphere where temperatures are actually higher.
So yes, there is lots of wavelength specific detail. Most of the radiation from 9 to 11 is from the surface except near the centre of that where Ozone plays a role - that is just visible on the above graph as a dip starting at the right extreme.
However, at a broader level, dealing with averages across wavelengths, the broad picture I have painted is still valid.
I prefer a graph which shows actual flux, not log of intensity, to get a more accurate visual image of what's going on, and one which covers Earth's entire upwelling IR spectrum of 7 microns to 100, not one artificially cut off at 10 and 25 microns. In this case we see a CO2 band of IR emitting at 220 K but that the majority of Earth's IR radiates from near the 300 K Planck curve, which means it's not being absorbed by any greenhouse gases. Yes I do know about the 9.6 micron O3 band which also radiates from higher elevations and lower temperatures.
graph
Earth's greenhouse atmosphere is like an actual greenhouse, with one section of glass representing CO2, another section for H2O vaor, and most of the roof wide open.
Mike Hillis
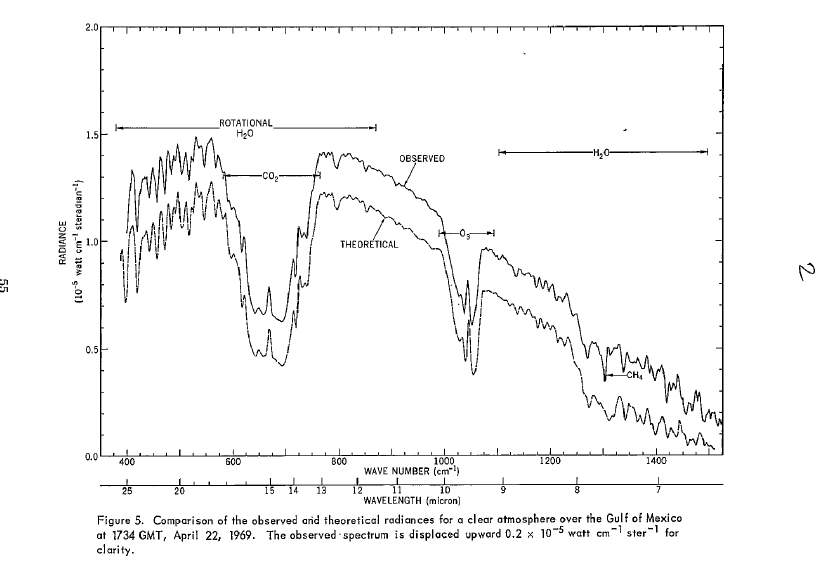
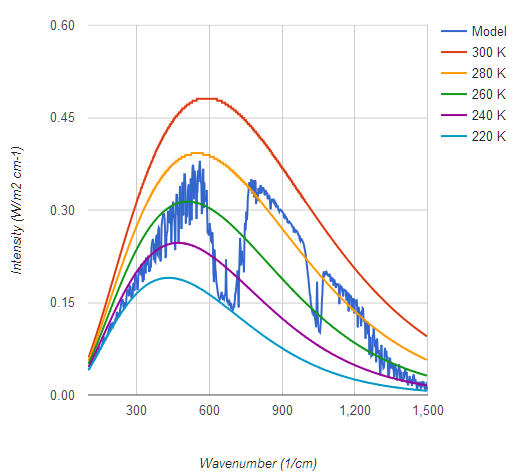
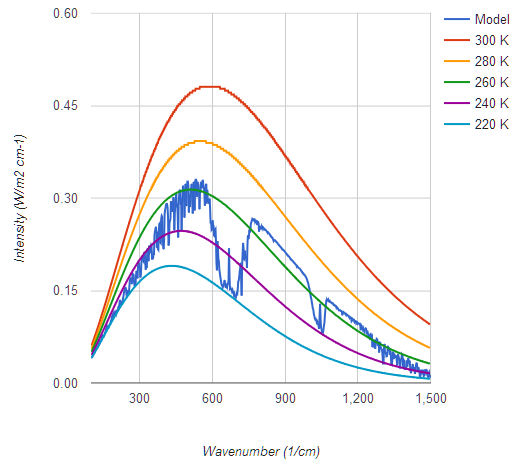
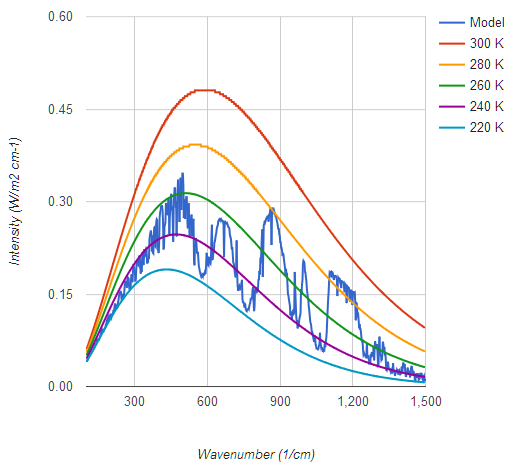
My graph is from Conrath et al 1970 - NASA used to host a non-pay-walled copy. To my knowledge this was the first time such a measurement was possible, from the Nimbus 3 satellite. This graph shows the corresponding planck curves. Figure 5 from the paper shows both theoretical and observed data from most of the pectrum, with a vertical displacement of one graph for clarity. Impressive agreement between theory and observation given it was 1969.
The cutoff above 25 micron is because that was the range of the instrument.
Regarding your graph, yep, that is a typical tropical profile.
Note how everything to the left of the CO2 notch, due to H2O Rotational absorption, is all substantially below the planck curve from the atmospheric window.
What is missing from this, since it is a clear sky simulation, is the effect of clouds. With clouds the curve sits significantly below the surface planck line across most of the spectrum. You can explore this here. at the Uni of Chicago - an online hosted copy of the Modtran simulator. You can select broad atmosphere profile, cloud type, gas concentrations and where you are looking from.
This is current gas composition, US Standard Atmosphere to be indicative of the entire planet, no clouds, looking down from 70km.
Here is the same set up but with a cumulus cloud base
As you can see, clouds modify the picture significantly. Here nothing is originating from the surface.
So with a reasonable proportion of the Earth cloud covered, on average, only a small percentage of surface radiation escapes directly from the surface.
An interesting exercise with the Chicago simulator is to select a configuration and progressively look down from lower and lower altitudes all the way to zero. Gives a sense of how high in the atmosphere different parts of the spectrum originate from.
This is a simulation of an atmosphere with 400,000 ppm. It isn't completely realistic since it still assumes a standard temperature profile, no extra water vapour and may not model continuum absorption, but as you can see, lots of extra effects and significantly less energy escaping to space..
Here's the famous Clough diagram showing the height of radiation of various bands. A major portion of the spectrum, from wave numbers 700 to 1300 waves/cm., are emitted from the surface. Plus a little more from 500 to 600. Come to think of it, it looks like Earth radiates from the surface in all but the tiny CO2 band from 600 to 700 and a little around ~300. Hmmmmm.
<Image data deleted>
[PS] Not sure what is going on here. You cannot embed an image. It has to be hosted somewhere else online. Try again.